Overview
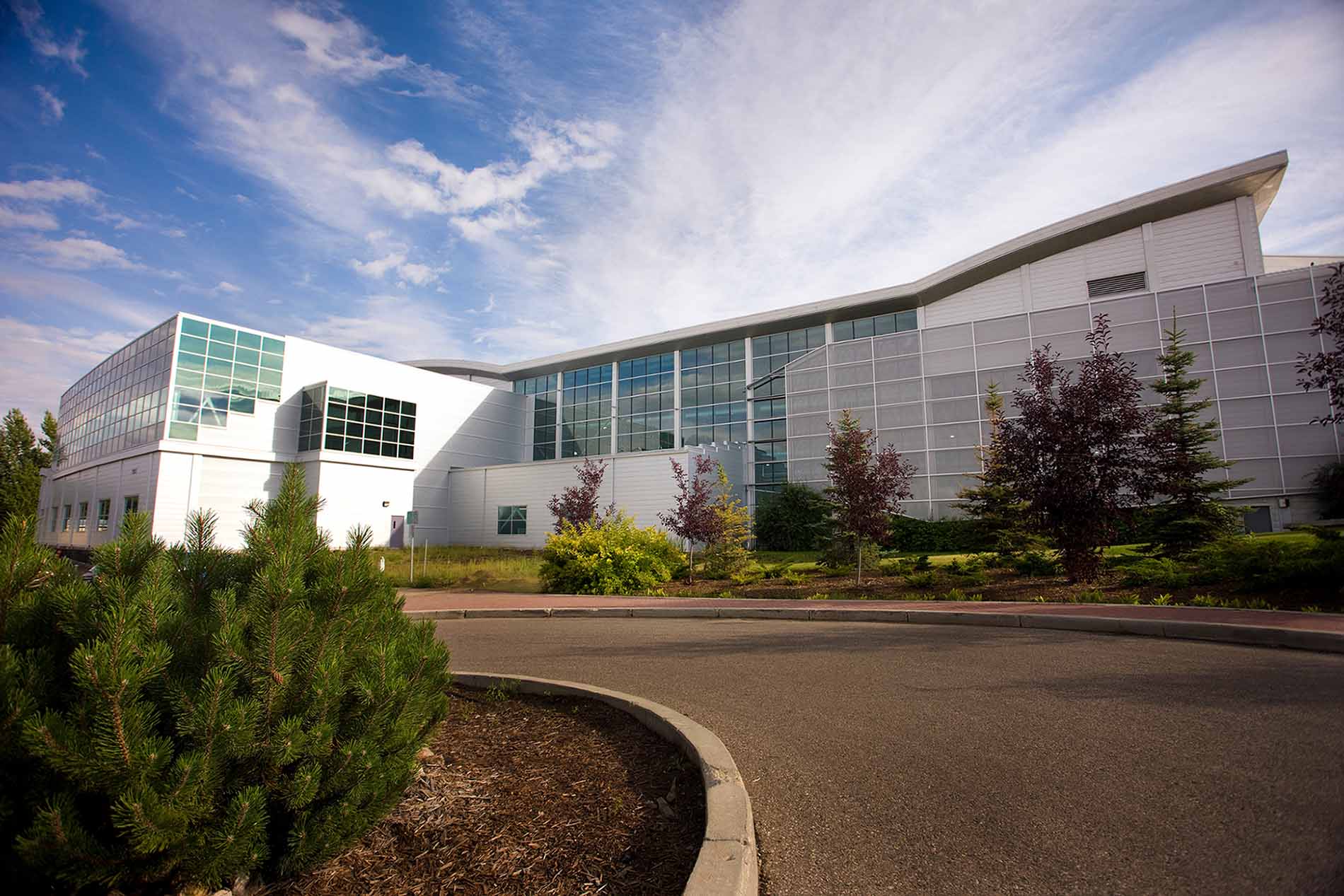
A synchrotron produces different kinds of light in order to study the structural and chemical properties of materials at the molecular level. This is possible by looking at the ways light interacts with the individual molecules of a material.
The CLS synchrotron produces light by accelerating electrons to nearly the speed of light and directing the electrons around a ring. The electrons are directed around the ring by a combination of radio frequency waves and powerful electromagnets. When the electrons go around the bends, they give off energy in the form of incredibly bright and highly focused light. Different types of light, primarily infrared and X-ray, are directed down to the end of beamlines, where researchers use the light for their experiments at endstations. Each beamline and endstation at the CLS is designed for a specific type of experiment.
Parts of the synchrotron
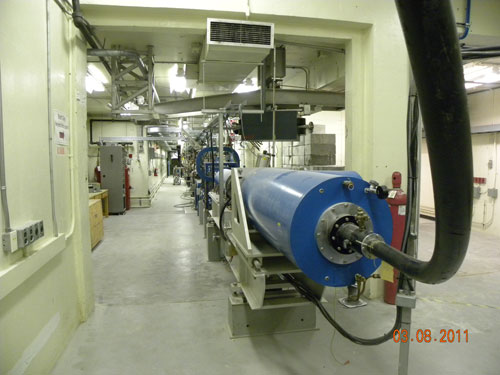
In the electron source, bursts of electrons are injected into an ultra-high vacuum stainless steel tube. These electrons will eventually create the synchrotron light that we use for research.
The electron source uses a tungsten button as the cathode. As electricity flows through the cathode, it becomes heated (at about 1000°C) to incandescence which gives some electrons enough energy to leave the surface of the cathode, essentially boiling electrons off. As this is happening, a nearby screen is given a short, strong positive charge which pulls the electrons away from the cathode towards the LINAC. (This process is similar to that found in a cathode ray tube (CRT) television). The high voltage electricity running through the cathode also repels the electrons being produced by the cathode and accelerates them towards the LINAC.
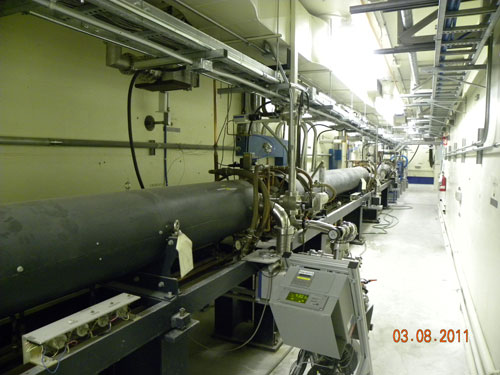
The linear accelerator, or LINAC, accelerates electrons in a straight line. Electrons produced by the electron gun enter the LINAC, where a series of radio frequency cavities with fields at 2,856 MHz accelerate the electrons to an energy of 250 million electron volts, or 250 MeV. At this energy the electrons are travelling at 99.9998% of the speed of light (3.0 x 108 m/s).
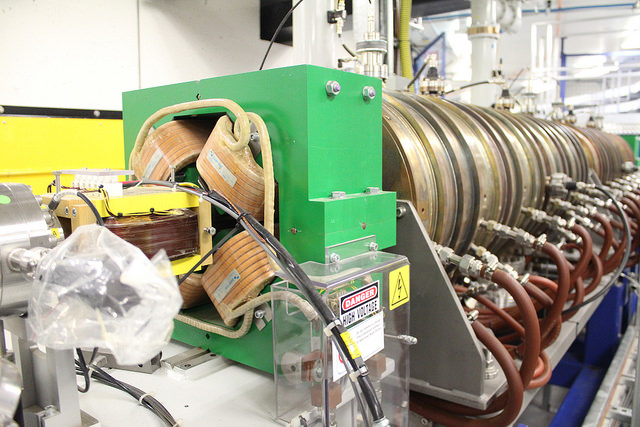
Electrons travel from the LINAC to the Booster Ring where a specifically-designed Radio Frequency (RF) cavity raises the energy of the electrons from 250 MeV to 2900 MeV as they circulate in the booster ring. Following this boost in energy, the electrons are transferred to the storage sing.
As electrons circulate the 103 m Booster Ring approximately 1.5 million times in 0.6 seconds, they receive a boost in energy from microwave fields generated in the RF cavity at 500 MHz to reach a total energy of 2900 MeV. How much energy is 2900 MeV? If we were to instead try to boost the electrons' energy using household batteries, it would take about 2 billion batteries connected in a row!
For comparison, the typical atmospheric molecule has an energy of about 0.03 eV and the energy of charged particles in a nuclear explosion can range from 0.3 to 3 MeV.
The booster ring cannot increase the speed of the electrons to, or beyond, the speed of light, but the electrons travel at about 99.999998% of the speed of light.
*In particle physics, the standard unit to measure energy is mega-electron volts or MeV which is 1*106 eV. One eV is the amount of energy that an electron gains when it moves through a potential difference of 1 volt in a vacuum.*
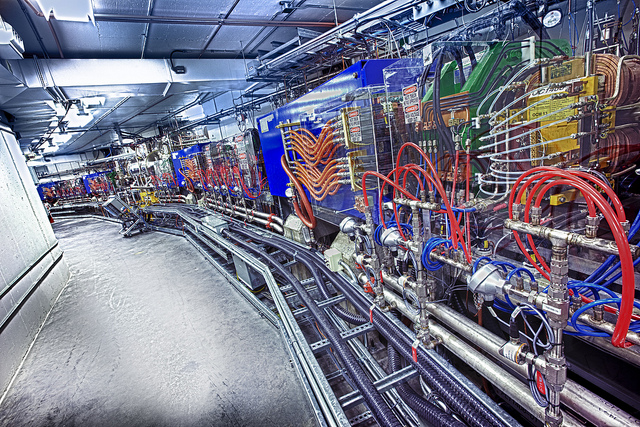
The high energy electrons from the booster ring are transferred into the storage ring when they reach 2900 MeV where they circulate for 4 to 12 hours, producing light for beamlines. The transfer process repeats once per second for up to 600 cycles (about 10 minutes), as required, to reach an average circulating current in the storage ring of 220 mA.
While the storage ring looks circular from the outside, it is really a series of 12 straight sections each with a series of magnets for bending the electrons' path and focusing the electron beam. Electrons normally travel in a straight line, so their path is bent by large (6800 kg) magnets at each turn, and this also causes the electrons to emit synchrotron light. There are also special magnetic arrays called insertion devices that produce a brighter light for certain beamlines.
Over time, the number of electrons stored in the ring will decline. This is inevitable because the vacuum isn’t perfect. Electrons collide with one another and the few particles that are present causing them to be lost. To maintain 220 mA of beam current, the CLS runs in Top-Up mode where a small amount of electrons are automatically injected every few minutes.
Everything from the electron gun to the beamlines is under vacuum. The electrons (and later the photons) must travel in a vacuum to avoid colliding into particles and disappearing. The storage ring vacuum chamber pressure is generally around 10^-10 torr. This means there are fewer than 10 particles per cubic centimeter present in our vacuum system.
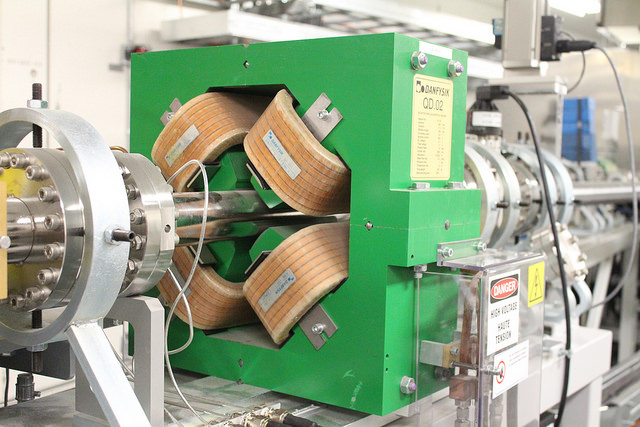
There are three main types of electro-magnets used at the CLS. There are the dipole magnets, the quadrupole magnets, and the sextupole magnets. The magnetic field created by the blue dipole (two-pole) magnets is used to direct the electrons around the booster and storage rings. The field of the green quadrupole (four-pole) and red sextupole (six-pole) magnets are used to force bunches of electrons into a fine beam within the vacuum chamber. The booster ring uses only dipole and quadrupole magnets, whereas the storage ring uses dipole, quadrupole, and sextupole magnets.
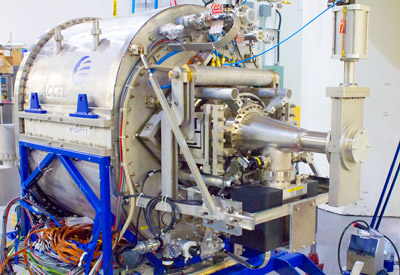
The CLS uses two radio frequency (RF) cavities - one in the booster ring and one in the storage ring- to boost the energy of circulating electrons using microwaves.
The booster ring has a cylindrical cavity that delivers a high-energy kick to the electron bunches during each turn around the ring. It operates with a RF of 500 MHz.
The storage ring uses an RF cavity to replace the energy lost by electrons as they produce light. The RF cavity in the storage ring is also superconducting. Superconductivity means that there is no resistance in the flow of electric current in certain metals and alloys at temperatures near absolute zero. The operating temperature of the storage ring RF cavity is -270°C (-273°C is absolute zero, the point at which all motion stops). Operating at such cold temperatures eliminates most power loss, while the RF field provides energy to the electrons.
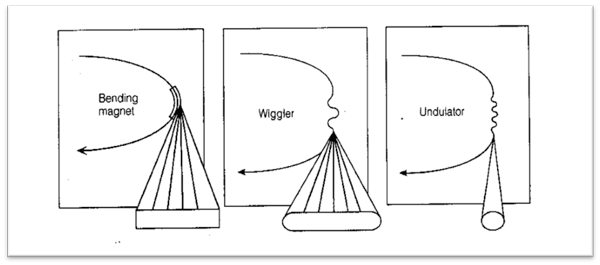
The CLS is one of the brightest synchrotrons in the world despite being one tenth the size of similarly bright synchrotrons. One of the ways that CLS achieves its brightness is through insertion devices. While dipole magnets change the direction of the electrons producing light, the multi-magnet insertion devices called wigglers and undulators placed in the straight section of the storage ring move or ‘wiggle’ electrons back and forth multiple times creating a narrow beam of highly intense light.
- A wiggler produces a wide range of high energy X-rays.
- An undulator produces even higher intensity X-rays with a narrower range of energies.
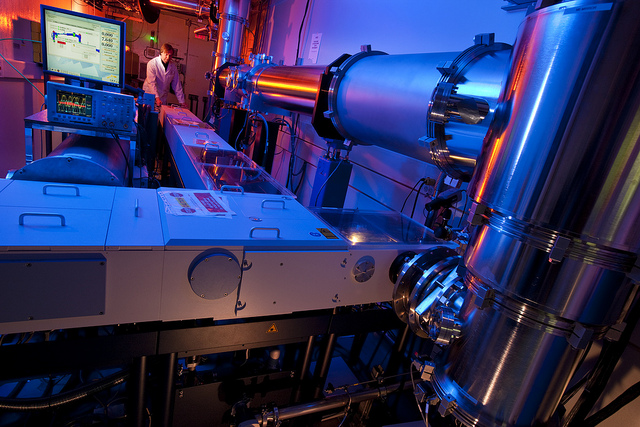
The CLS has 22 beamlines accessible to scientists using the synchrotron as part of their research. A beamline consists of an optics hutch where synchrotron light is focused and wavelength is selected, an experimentation hutch where the appropriate technique is selected for the experiment, and work stations where scientists operate the beamline and measure light as it is absorbed, reflected, refracted, or scattered by the sample.
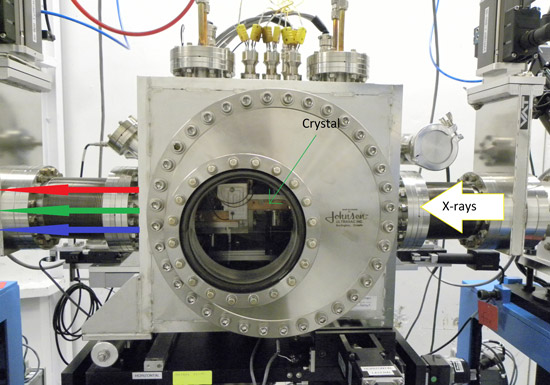
At the beamline, the synchrotron light passes through the optics hutch on its way to the sample. There, the monochromator enables researchers to choose the wavelength of light best-suited to the experiment they are conducting. The monochromator is the device that separates different wavelengths of light (much like a prism). This is done using either optical dispersion (as in a prism), or as diffraction, using a grating which separates the wavelengths of light and filters out the light that isn’t required. Each of the beamlines at CLS is unique and have markedly different monochromators specific to their design.
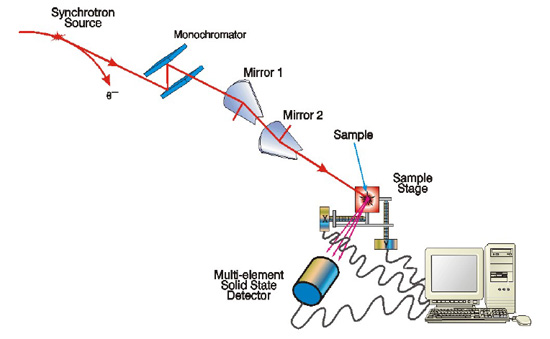
The selected wavelengths of synchrotron light are focused by the mirrors in the optics hutch onto the sample in an endstation located in the experimental hutch. Each endstation is designed specifically for the types of experiments conducted on that beamline. In general, each one consists of a sample holder and a detection system, as well computers through which the researchers control their experiments and view data as it is recorded.
About Synchrotron Light
When a synchrotron accelerates electrons, they are "bent" around corners using magnets. When the particles go around the corners they release photons, or little bits of all kinds of light. We can then filter out the spectra, or kinds, of light we want such as Infrared (IR), Ultra-Violet (UV), and X-Ray light.
Though a synchrotron is not the only way to generate IR, UV or X-Ray light, we experience substantial benefits in brightness, experiment quality and speed, along with increased ability to select specific light wavelengths. Synchrotron light is emitted when the path of an electron beam is altered via extremely powerful magnets.
Radiation is energy that comes from, or radiates from, a source and travels through material or through space. Sources of radiation include light, heat and sound. There are many natural sources of radiation, including the sun and various elements in the earth.
At CLS, radiation is mainly used as another word for light. Often, people use the word radiation instead of light when they are talking about the wavelengths, or types, of light that the human eye can't see, like UV light from the sun.
If you were to expose a 1 mm x 1mm sample, similar to what a researcher might put under a regular light microscope, to a number of different light sources and measure the amount of energy the matter in that sample interacted with, you would find that the energy generated by a synchrotron using insertion devices is considerably higher than what is produced by other light sources.
Generally speaking, synchrotron sources pack more photons into a smaller beam of light. This offers researchers more information about their sample and makes a greater variety of techniques available to use to learn about their sample.
Another advantage to some synchrotron techniques is the ability to conduct experiments in situ, or as they are – without treatment. There are a number of research techniques that require the scientist to treat their sample (crush it; make a solution; slice it; etc). While this is also required for some synchrotron techniques, there are also some that allow for the sample to be analyzed without treatment or with less treatment, which can be a significant advantage.
Tuneability is the ability to select a specific kind of light.
By producing high flux light across a significant portion of the spectrum, a synchrotron offers many different techniques to researchers in one building. In order to gather information, the wavelength of the light has to be appropriate for the size of the matter of interest. Shorter wavelengths allow scientists to gather information about smaller things.
In addition, each element absorbs energy at a known level. Being able to select a specific wavelength, or range of wavelengths, allows researchers the flexibility to direct their research towards specific questions.
Due to the extreme brightness of the light, it does not take as long to conduct the same experiment using a synchrotron source of light as it does with a ‘table top’ source for some techniques.
Uses for a Synchrotron
A synchrotron can be used to probe matter and analyze many physical, chemical, geological, and biological processes. Information obtained by scientists from these analyses can be used to help design new medications, build next-generation technology, examine surfaces and materials to make more effective products like motor oils, develop new materials for safer medical implants, help with the clean-up of mining wastes, and find innovative ways to combat climate change to name just a few applications.
For the general public, the main purpose of a synchrotron is the research news that is published and the eductional programs for students and educators that are offered.
For scientists, the main purpose of a synchrotron is research. The different areas of research that the CLS synchrotron conducts can be split into 4 categories of technical information, each with their own subcategories.
CLS scientists work with industrial teams to develop an experimental plan based on the client’s needs, providing key answers to critical questions. Learn more about the opportunities for industry professionals at the CLS.
Are Synchrotrons Safe?
The CLS is committed to providing a safe working environment for all staff and protecting the general public and environment from risks. As part of our commitment to safety, we utilize personnel safety systems, equipment protection systems and emergency shutdown processes.
Click through the list to learn about our safety protocols.
For more specific questions, including things like "What if someone gets hit by a beamline?", check out our Safety FAQs page.
Control Room management is an important part of ensuring personnel safety throughout the facility. From the control room we monitor:
- Personnel Safety Systems (PSS) - includes Active Area Radiation Monitor System, Fire Alarms System, Accelerator Access Interlock Systems, Oxygen Monitoring System
- Equipment Protection Systems (EPS) - includes beam position, size and quality, cooling/heating systems, vacuum systems, power supplies and magnet settings, timing systems, and valve controls
- Accelerator operators disable/enable all or individual beamlines including start and shutdown processes and select mode of accelerator operation such as normal (beam available to users) versus accelerator studies
In the case of an emergency the synchrotron can be shut down automatically in less than 20 milliseconds from the control room and from several other locations manually.
Because the Canadian Light Source uses a high-energy electron accelerator to make synchrotron radiation, a licence to operate from the Canadian Nuclear Safety Commission (CNSC) is required. Some radiation produced at a synchrotron facility can be harmful. Protection of workers and scientists at the synchrotron starts with the facility design. Thick concrete walls contain radiation produced by the electron beam, and the synchrotron radiation used for science is contained within lead- and steel-lined rooms. A safety system keeps people out of these areas when the beam is on, and turns the beam off instantly if someone attempts to enter. Radiation levels in areas where someone can be present are constantly measured in different ways to ensure radiation exposures to workers and scientists are kept well below the CNSC regulatory limits. No one at the Canadian Light Source has ever received a radiation exposure greater than the CNSC public limit.
- Typical Annual Exposure to CLS Worker: < 0.1 mSv
- Typical Exposure from a chest X-Ray: ~0.1 mSv (millisievert)
- CNSC Annual Public Exposure Limit: 1 mSv
- Natural Background Radiation in Canada: ~3 mSv
- CNSC Nuclear Energy Worker Annual Limit: 50 mSv
Learn More
Students and Educators
CLS offers a variety of short- and long-term programming for students as well as educators from across Canada. Learn more about getting synchrotron science into the classroom.
Academic Researchers
Academic clients can submit proposals through a peer review process, get access to beam time, and more. Learn more about individual beamlines and techniques offered by CLS.
Industry Professionals
CLS scientists work with industrial teams to develop an experimental plan based on the client’s needs, providing key answers to critical questions. Learn more about the opportunities for industry professionals at the CLS.
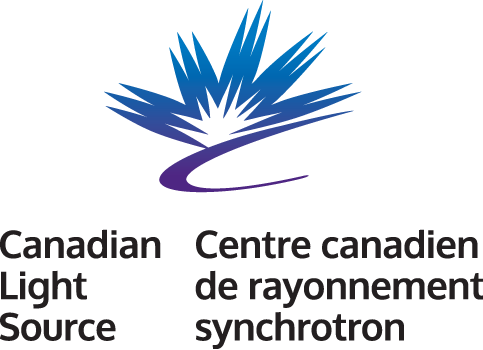